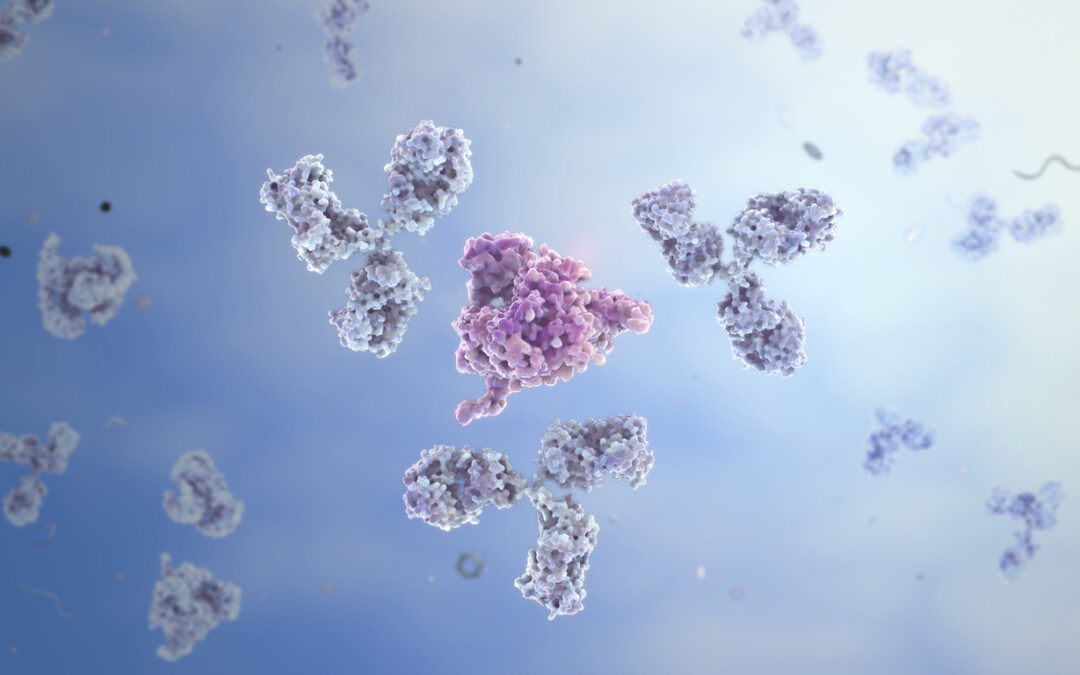
NAD+ Peptide and Cellular Aging
NAD+ exists in two forms: oxidized (NAD+) and reduced (NADH). The scientific theory posits that the oxidized form, NAD+, accepts electrons during reactions, while the reduced form, NADH, donates electrons. This interconversion between NAD+ and NADH may allow for the transfer of energy and electrons between molecules in metabolic pathways.
Nicotinamide Adenine Dinucleotide appears to participate in several essential metabolic processes, including cellular respiration, DNA repair, redox reactions, and metabolic regulation. NAD+ may be a crucial player in glycolysis, the Krebs cycle (citric acid cycle), and oxidative phosphorylation. During these processes, NAD+ may accept electrons and become reduced to NADH, possibly carrying the electrons to the electron transport chain for ATP production. NAD+ appears also to be involved in regulating several other metabolic pathways, including lipid metabolism and the biosynthesis of macromolecules.
Furthermore, NAD+ may be involved in DNA repair mechanisms, particularly in a class of enzymes called sirtuins. Sirtuins are considered to utilize NAD+ as a cofactor to remove acetyl groups from proteins, possibly regulating their activity and influencing DNA repair, gene expression, and cellular longevity.
NAD+ Peptide and Cellular Longevity
Researchers posit NAD+’s potential in various processes related to cellular longevity, particularly in DNA repair and gene expression regulation through sirtuins.[1] Sirtuins are a class of proteins that are considered to require NAD+ as a cofactor to carry out possible enzymatic activities. They are potentially involved in various cellular processes, including DNA repair, gene expression, and metabolic regulation. They have been linked to cellular longevity and lifespan.[2] The Sirtuin family of enzymes, such as SIRT1, appear to be NAD+-dependent protein deacetylases and may have the potential to sense NAD+ levels. Scientific reviews hypothesize that sirtuins may primarily sense NAD+ not NADH or the NAD+/NADH ratio.[3] However, the mechanisms by which enzymes like sirtuins respond to cell redox changes are not fully understood.
Researchers have proposed that the decline in NAD+ levels that may occur with aging might contribute to age-related cellular dysfunction, and restoring or maintaining NAD+ levels might potentially mitigate it. For example, one experiment in worms suggested that NAD(+) levels decline with age and that reducing NAD(+) may further decrease lifespan.[4] Conversely, restoring NAD(+) through genetic or other means appeared to promote longevity in worms. The researchers hypothesized that these actions might rely on the activity of the SIRT1, otherwise known as Protein Deacetylase Sir-2.1 or Silent Information Regulator 2.1, and may involve the induction of imbalances in mitochondrial proteins, as well as the activation of stress signaling pathways like the mitochondrial unfolded protein response (UPR(mt)) and the nuclear translocation and activation of the FOXO transcription factor DAF-16. The authors also considered that enhancing mitochondrial stress signaling by modulating NAD(+) levels might be a potential strategy to support mitochondrial function.
NAD+ Peptide and Cellular Respiration
Researchers should note that “Nicotinamide adenine dinucleotide (…) is a coenzyme for redox reactions, making it central to energy metabolism.“[5] NAD+ may play a crucial role in cellular respiration, the process by which cells convert nutrients into usable energy. Cellular respiration is considered to occur in multiple steps involving different metabolic pathways, and NAD+ has been posited to be involved in two specific stages: glycolysis and the citric acid cycle (also known as the Krebs cycle or tricarboxylic acid/TCA cycle).[6]
Scientists consider that glycolysis, the process by which glucose is broken down into pyruvate, may generate a small amount of ATP (adenosine triphosphate) and NADH. Researchers posit that Nicotinamide Adenine Dinucleotide accepts electrons and a hydrogen ion (H+) from glucose, forming NADH. NADH may carry these high-energy electrons to the next stage of cellular respiration. In the citric acid cycle, the pyruvate produced in glycolysis is considered to break down further, potentially releasing additional energy. NAD+ appears to be involved in several reactions, possibly t accepting electrons and H+ ions, forming NADH. These reactions appear to occur within the mitochondria, the cell’s energy-producing organelles.
NADH that appears to be generated in both glycolysis and the citric acid cycle, has the potential to carry the high-energy electrons to the electron transport chain, the final stage of cellular respiration. Here, NADH may donate its electrons to the chain, allowing them to flow through a series of protein complexes. This electron flow appears to drive protons (H+) pumping across the mitochondrial membrane, potentially establishing an electrochemical gradient. Eventually, the electrons and protons may combine with oxygen to form water, while the energy released by the electron flow may produce ATP through oxidative phosphorylation. As NADH donates its electrons to the electron transport chain, it may be converted back to NAD+, ready to possibly participate in the next round of glycolysis and the citric acid cycle. This regeneration of NAD+ may be instrumental in sustaining possible continuous production of ATP through cellular respiration.
NAD+ Peptide and DNA Repair
One of the key enzymes researched in connection to DNA repair, poly(ADP-ribose) polymerase (PARP), appears to rely on NAD+. When DNA damage occurs, PARP may possibly be activated, potentially bindingto the damaged site on the DNA. PARP may utilize NAD+ as a substrate to add ADP-ribose units to itself and other target proteins, a process termed poly(ADP-ribosylation) by researchers.[7] This modification has the potential to help recruit and activate other DNA repair proteins, possibly facilitating the repair of DNA lesions.
The poly(ADP-ribosylation) process appears to generate poly(ADP-ribose) (PAR) chains, which may serve as a signal for DNA repair machinery to recognize and target damaged DNA. PARP may also play a role in recognizing and repairing single-strand breaks (SSBs) in DNA. Should NAD+ be a cofactor, PARP may help maintain genomic stability by initiating and coordinating DNA repair processes. However, this process may also deplete cellular NAD+ levels, which may impact other NAD+-dependent cellular processes, such as energy metabolism and signaling. Studies comment that “in response to DNA damage, the rate of PAR synthesis increases rapidly up to 500-fold, which can consume a significant amount of NAD+.“[8] Thus, the researchers hypothesize that the depletion of intracellular NAD+ due to PARP1 activation may affect the NAD+/SIRT1 axis, possibly leading to defects in mitochondrial homeostasis, ROS (reactive oxygen species) production, DNA repair, and cell survival.
Conclusion
In conclusion, Nicotinamide Adenine Dinucleotide has been suggested by researchers to act as a crucial coenzyme involved in various biological processes, particularly cellular metabolism. It might participate in essential metabolic pathways, such as cellular respiration, DNA repair, and metabolic regulation. NAD+ has been suspected to play a key role in energy production through glycolysis and the citric acid cycle, where researchers suggest it may accept electrons and transfer them to the electron transport chain for ATP synthesis. Additionally, NAD+ appears to be involved in DNA repair mechanisms, specifically through enzymes like sirtuins and PARP, which possibly utilize NAD+ peptide to regulate gene expression, cellular longevity, and genomic stability.
Disclaimer: The products mentioned are not intended for human or animal consumption. Research chemicals are intended solely for laboratory experimentation and/or in-vitro testing. Bodily introduction of any sort is strictly prohibited by law. All purchases are limited to licensed researchers and/or qualified professionals. All information shared in this article is for educational purposes only.
References
- Imai, S., & Guarente, L. (2014). NAD+ and sirtuins in aging and disease. Trends in cell biology, 24(8), 464–471. https://doi.org/10.1016/j.tcb.2014.04.002
- Wątroba, M., Dudek, I., Skoda, M., Stangret, A., Rzodkiewicz, P., & Szukiewicz, D. (2017). Sirtuins, epigenetics and longevity. Aging research reviews, 40, 11–19. https://doi.org/10.1016/j.arr.2017.08.001
- Anderson, K. A., Madsen, A. S., Olsen, C. A., & Hirschey, M. D. (2017). Metabolic control by sirtuins and other enzymes that sense NAD+, NADH, or their ratio. Biochimica et biophysica acta. Bioenergetics, 1858(12), 991–998. https://doi.org/10.1016/j.bbabio.2017.09.005
- Mouchiroud, L., Houtkooper, R. H., Moullan, N., Katsyuba, E., Ryu, D., Cantó, C., Mottis, A., Jo, Y. S., Viswanathan, M., Schoonjans, K., Guarente, L., & Auwerx, J. (2013). The NAD(+)/Sirtuin Pathway Modulates Longevity through Activation of Mitochondrial UPR and FOXO Signaling. Cell, 154(2), 430–441. https://doi.org/10.1016/j.cell.2013.06.016
- Covarrubias, A. J., Perrone, R., Grozio, A., & Verdin, E. (2021). NAD+ metabolism and its roles in cellular processes during ageing. Nature reviews. Molecular cell biology, 22(2), 119–141. target=”_blank” rel=”noopener”https://doi.org/10.1038/s41580-020-00313-x
- Ahmad M, Wolberg A, Kahwaji CI. Biochemistry, Electron Transport Chain. [Updated 2022 Sep 5]. In: StatPearls [Internet]. Treasure Island (FL): StatPearls Publishing; 2023 Jan-. Available from: https://www.ncbi.nlm.nih.gov/books/NBK526105/
- Leung, A., Todorova, T., Ando, Y., & Chang, P. (2012). Poly(ADP-ribose) regulates post-transcriptional gene regulation in the cytoplasm. RNA biology, 9(5), 542–548. https://doi.org/10.4161/rna.19899
- Croteau, D. L., Fang, E. F., Nilsen, H., & Bohr, V. A. (2017). NAD+ in DNA repair and mitochondrial maintenance. Cell cycle (Georgetown, Tex.), 16(6), 491–492. https://doi.org/10.1080/15384101.2017.1285631