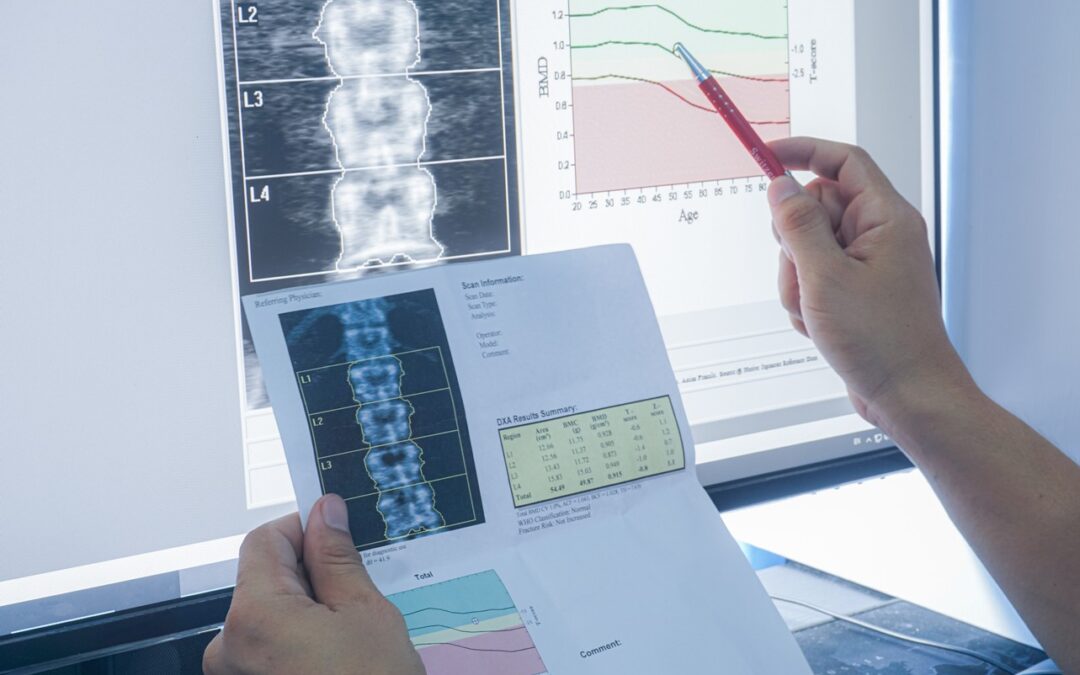
Studies in Ipamorelin for Muscle Growth and Bone Density
Ipamorelin peptide appears to differentiate itself from other GHSs as a potentially more selective option that elevates GH levels without increasing other pituitary hormones, such as prolactin. Studies are still underway with this peptide within the context of postoperative ileus and speeding up the recovery of gastrointestinal function following damage.
Research
Ipamorelin and Growth Hormonse Synthesis
Studies suggest that Ipamorelin may be a highly selective growth hormone secretagogue, which may be capable of increasing GH levels in animals by activating the GHS-R receptors.[1,2]
Research studies report that the effect may occur relatively quickly – as soon as 40 minutes after exposure, there appeared to be a peak in GH levels. Increasing GH levels may potentially have numerous potential impacts, such as preserving muscle and lean body mass, increasing energy levels, improving bone mineral density, and more. [3]
Ipamorelin and Gastrointestinal Functions
Ipamorelin has been studied for its potential to alleviate delayed gastric emptying and post-surgical ileus in animals. In rodents, the peptide may significantly accelerate the rate of gastric emptying through stimulating gastric contractility.[4] The route via which the peptide may act appears to be by activating a ghrelin receptor-mediated mechanism involving cholinergic excitatory neurons. The researchers reported that “Ipamorelin (0.014 µmol/kg intravenous) resulted in a significant acceleration (P < 0.05 vs vehicle-treated rat) of gastric emptying with 52% ± 11% of the meal remaining in the stomach compared to nonsurgical control animals with 44% ± 6%.”
Ipamorelin and Appetite, Weight
Existing research suggests that Ipamorelin may increase appetite, reduce weight loss in the context of research studies in wasting disorders. This hypothesis is based on animal study findings. According to the researchers, these hypotheses are due to the apparent appetite-increasing characteristics of Ipamorelin.[5] The peptide appears to activate the receptors of the hunger hormone, which in turn may result in an increased food intake.
On the other hand, any growth hormone-increasing potential of Ipamorelin may also help reduce weight loss, especially protein loss. Studies posit that Ipamorelin may reduce muscle wasting in cortisol-exposed animals and help maintain a positive nitrogen balance.[6] The researchers conclude that “Accelerated nitrogen wasting in the liver and other organs caused by prednisolone [exposure] was counteracted by [influence] with either GH or its secretagogue Ipamorelin.”
Another trial observes that Ipamorelin appears to negate the GH-inhibiting action of glucocorticoids in tested animals.[7] Animal studies suggest that Ipamorelin may also stimulate insulin secretion, another anabolic hormone that can help reduce muscle loss in wasting disorders.[8]
Ipamorelin and Bone
Growth hormones are considered a factor in maintaining optimal bone mineral density. Animal studies suggest that thanks to Ipamorelin’s alleged action on growth hormone synthesis and body weight, it may help maintain or even increase bone mass. One study on thirteen-week-old female Sprague-Dawley rats suggested that Ipamorelin exposure appeared to significantly increase bone mineral content after 12 weeks.[9] The increase was measured via a DEXA (dual-energy X-ray absorptiometry) scan and was significantly higher than the placebo group.
Another animal trial on rats treated with glucocorticoids reported that Ipamorelin has the potential to completely negate bone loss induced by glucocorticoids.[10] The scientists report that the periosteal bone formation rate increased four-fold in animals exposed to glucocorticoids and Ipamorelin in combination, compared with the group that received glucocorticoids alone.
Disclaimer: The products mentioned are not intended for human or animal consumption. Research chemicals are intended solely for laboratory experimentation and/or in-vitro testing. Bodily introduction of any sort is strictly prohibited by law. All purchases are limited to licensed researchers and/or qualified professionals. All information shared in this article is for educational purposes only.
References
- Raun K, Hansen BS, Johansen NL, Thøgersen H, Madsen K, Ankersen M, Andersen PH. Ipamorelin, the first selective growth hormone secretagogue. Eur J Endocrinol. 1998 Nov;139(5):552-61. doi: 10.1530/eje.0.1390552. PMID: 9849822.
- Gobburu JV, Agersø H, Jusko WJ, Ynddal L. Pharmacokinetic-pharmacodynamic modeling of ipamorelin, a growth hormone releasing peptide, in human volunteers. Pharm Res. 1999 Sep;16(9):1412-6. doi: 10.1023/a:1018955126402. PMID: 10496658.
- Beck DE, Sweeney WB, McCarter MD; Ipamorelin 201 Study Group. Prospective, randomized, controlled, proof-of-concept study of the Ghrelin mimetic ipamorelin for the management of postoperative ileus in bowel resection patients. Int J Colorectal Dis. 2014 Dec;29(12):1527-34. doi: 10.1007/s00384-014-2030-8. Epub 2014 Oct 21. PMID: 25331030.
- Greenwood-Van Meerveld B, Tyler K, Mohammadi E, Pietra C. Efficacy of ipamorelin, a ghrelin mimetic, on gastric dysmotility in a rodent model of postoperative ileus. J Exp Pharmacol. 2012 Oct 19;4:149-55. doi: 10.2147/JEP.S35396. PMID: 27186127; PMCID: PMC4863553.
- Lall S, Tung LY, Ohlsson C, Jansson JO, Dickson SL. Growth hormone (GH)-independent stimulation of adiposity by GH secretagogues. Biochem Biophys Res Commun. 2001 Jan 12;280(1):132-8. doi: 10.1006/bbrc.2000.4065. PMID: 11162489.
- Aagaard NK, Grøfte T, Greisen J, Malmlöf K, Johansen PB, Grønbaek H, Ørskov H, Tygstrup N, Vilstrup H. Growth hormone and growth hormone secretagogue effects on nitrogen balance and urea synthesis in steroid treated rats. Growth Horm IGF Res. 2009 Oct;19(5):426-31. doi: 10.1016/j.ghir.2009.01.001. Epub 2009 Feb 23. PMID: 19231263.
- Malmlöf K, Johansen PB, Haahr PM, Wilken M, Oxlund H. Methylprednisolone does not inhibit the release of growth hormone after intravenous injection of a novel growth hormone secretagogue in rats. Growth Horm IGF Res. 1999 Dec;9(6):445-50. doi: 10.1054/ghir.1999.0128. PMID: 10629165.
- Adeghate E, Ponery AS. Mechanism of ipamorelin-evoked insulin release from the pancreas of normal and diabetic rats. Neuro Endocrinol Lett. 2004 Dec;25(6):403-6. PMID: 15665799.
- Svensson J, Lall S, Dickson SL, Bengtsson BA, Rømer J, Ahnfelt-Rønne I, Ohlsson C, Jansson JO. The GH secretagogues ipamorelin and GH-releasing peptide-6 increase bone mineral content in adult female rats. J Endocrinol. 2000 Jun;165(3):569-77. doi: 10.1677/joe.0.1650569. PMID: 10828840.
- Andersen NB, Malmlöf K, Johansen PB, Andreassen TT, Ørtoft G, Oxlund H. The growth hormone secretagogue ipamorelin counteracts glucocorticoid-induced decrease in bone formation of adult rats. Growth Horm IGF Res. 2001 Oct;11(5):266-72. doi: 10.1054/ghir.2001.0239. PMID: 11735244